Neutrinos play an exceptional role in particle and nuclear physics as well as in astrophysics. Postulated by Pauli in 1930 and named by Fermi in 1933, they were experimentally discovered by Reines and Cowan in 1956. Since then, new characteristics about their nature have been studied and discovered. Neutrinos [1] are found in sets of three leptonic flavours, namely electronic, muonic and tauonic [2].
Neutrinos interact through the electroweak theory [3] in interactions mediated by these electroweak vertices:
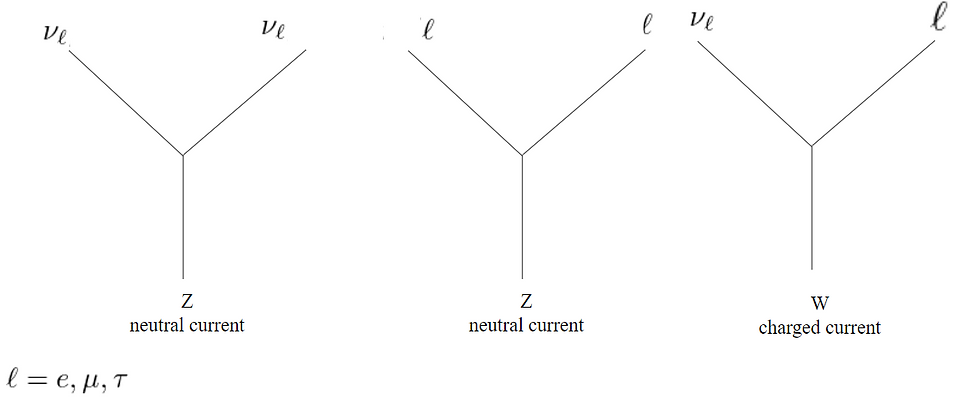
Nevertheless, we cannot be see neutrinos in experiments … but we can obtain the products of their interactions. Therefore, when we study neutrinos the vertex that we will be looking for is the one with the charged current, where a neutrino converts into a lepton.
KM3NeT (Cubic Kilometre Neutrino Telescope) is a next generation neutrino telescope, earlier generations of this experiments such as Antares and IceCube have already been shut down. Nontheless, KM3NeT is being build right now in two different locations in the deep Mediterranean sea. In the ARCA telescope array, KM3NeT scientists will search for neutrinos from distant astrophysical sources such as supernovae, gamma ray bursters or colliding stars. The ORCA telescope is the instrument for KM3NeT scientists studying neutrino properties exploiting neutrinos generated in the Earth's atmosphere.
These neutrino telescopes are composed of a series of arrays of thousands of optical sensors that will detect the faint light in the deep sea from charged particles originating from collisions of the neutrinos and the Earth. The experiment will also permit the study for Earth and Sea sciences for long-term and on-line monitoring of the deep sea environment and the sea bottom at depth of several kilometers.
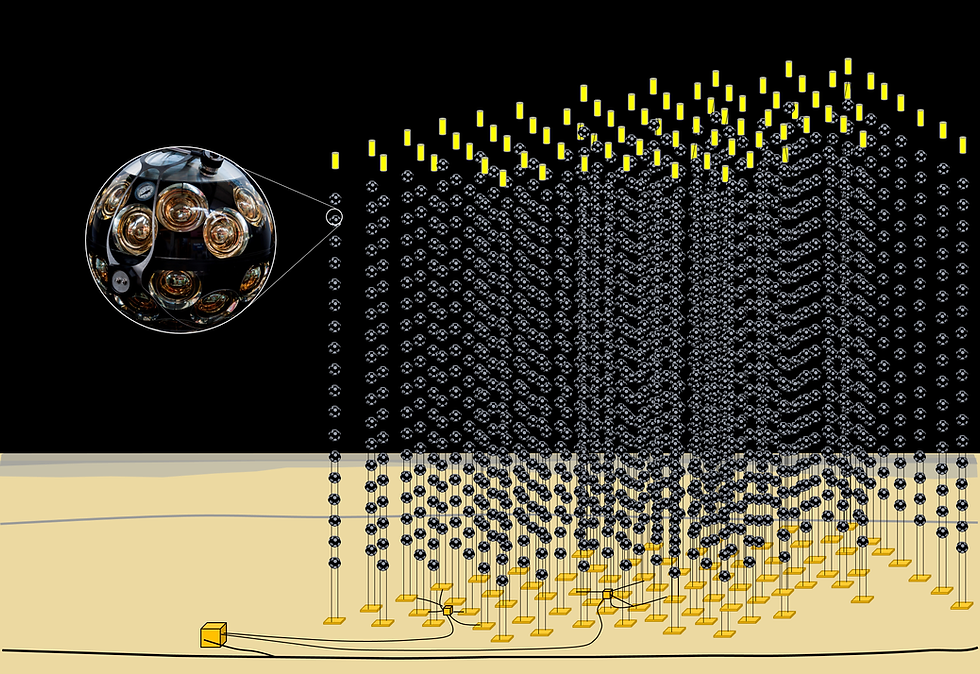
The interactions that involve neutrinos that we can search for in KM3NeT are those in which a neutrino converts into a lepton (electron, muon or tauon) [4] or vice versa. But, why are we so interested in looking for neutrinos?
Neutrinos interact very weakly with any kind of matter, they can cross the Earth bearly interacting with any particle. Neutrinos can, thus, travel long distances retaining all the information from the source where they were generated. There are very powerful events taking place in our universe, such as supernovae explosions and also nuclear reactions inside stars, that produce neutrinos. Since they travel undisturbed in straight lines directly from the source, we could recover the information from the source if we were able to reconstruct their paths and energies. This would give useful information about the way that our Universe works.
As I already made clear, we cannot measure the weak interaction in experiments… but we can measure electromagnetic interaction [5] (light). Leptons (electron, muon and tauon) are charged particles that interact with light (photons) throught the electromagnetic interaction. Particles cannot travel at speeds above the speed of light v=c=3x10^8 m/s in vacuum [6]; leptons are charged particles, and as such, when they enter a medium (water) they can travel at superluminic speeds v>c [7].

As leptons travel at superluminic velocities, they excite the water molecules to higher energy states; these molecules then, return to their fundamental states through the emission of light (photons). The photons that come from this desexcitation travel at velocities smaller that the speed of light v<c, creating an unusual blue light that we call Cherenkov radiation.
There will be electromagnetic interactions that happen between the charged particle in motion and the particles that are making up the medium it’s travelling through. Those interactions will cause the traveling charged particle to emit radiation in a particular energy in all allowable directions: radially outward, perpendicular to the direction of motion.
But since the particle emitting the radiation is in motion and moving quickly, all of those emitted photons are going to be boosted. Instead of getting a ring of photons that simply moves outward, this particle will emit a cone of radiation that travels in the same direction of motion as the particle emitting it. The Cherenkov radiation will come out with an angle defined by: the speed of the particle (moving faster than light in the medium but slower than light in vacuum) and the speed of light in the medium.
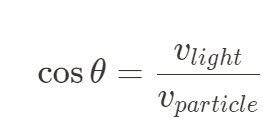
The angle of the Cherenkov radiation will allows us to determine the speed of the particle that caused its emission. If we measure Cherenkov light that originates from a particular particle, we can reconstruct that particle's properties.
Leptons leave very different signals in the KM3NeT arrays, as you can see in the image below. The most interesting signals are left by muons, because their reconstructed track is easier to obtain. In KM3NeT, we will usually be working with muonic reconstructed traks.
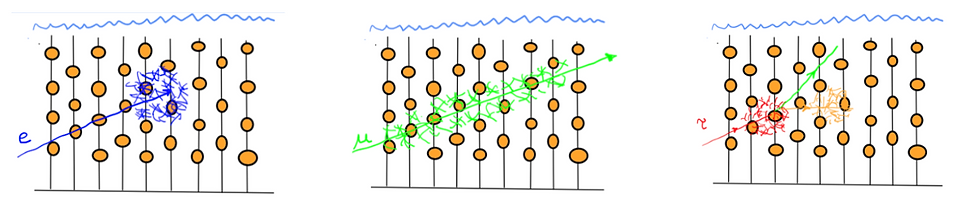
When we work with muons, we will find that most of the muons that arrive in the detector come from cosmic rays [8]. Therefore, we will need to make a cut and exclude all of the muons that can fall directly on the detector. Then, we will be left with muons produced from neutrinos (that cross the earth, unlike atmospheric muons).

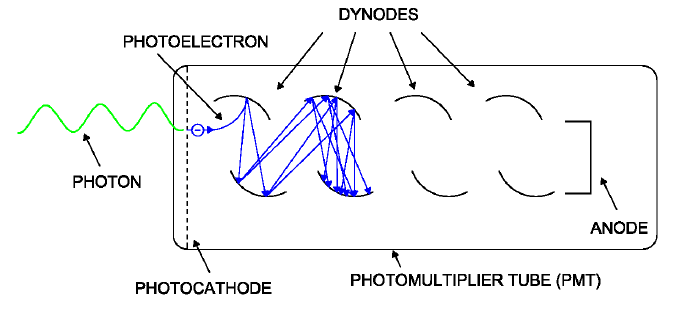
The signal that the muon leaves in the optical modules in the array will allow the reconstruction of the path and the energy from the muon. Although the light signal from the muon might be small, the photomultipliers inside of the optical modules will transform this light signal into an electrical signal and amplify it.
There is no infinite storage at the array, so after receving the information the array must decide if it is a signal or noise. For this there will be several prepared “triggers”, triggers are a set of rules that apply when the optical modules are activated. Depending on the combination of the activated optical modules, the array will save the data if it is considered a signal or delete it if the combination means noise.
After the appropiate measurements, the data will be saved in very complicated ways and recovered by scientists. The information for the angle of incidence, the timing, the energy, … of the incident particle will be stored and made availabe for scientists at KM3NeT collaboration to analyse. The analysis that can be made on the data cover wide extensions of Physics Fields. They can be used to study astrophysical sources, the oscillation of solar neutrinos, … and also the study of exotic particles such as Dark Matter.
---------------------------------------------------------------------------------------------------------------------------
Vocabulary:
[1] Neutrinos: elementary neutral particles from the Standard Model of Particle Physics that barely interact with matter. They can travel long distances undisturbed and retain all of the information from the original source. However, as they interact so weakly, they are very difficult to measure.
[2] Neutrino flavours: Neutrinos can be found in sets of three different flavours: electronic, muonic and tauonic. In the end, the flavour is a further description of the particle itself.
[3] Electroweak theory: In Particle Physics, the electroweak interaction is a unified description of thwo of the fours known fundamental interactions of nature: electromagnetism and weak interaction.
[4] Leptons: elementary charged particles from the Standard Model of Particle Physics that interact through the electromagnetic and the weak forces with other particles. We can find three kinds of leptons: electrons, muons and tauons.
[5] Electromagnetic interaction: It is one of the four fundamental forces of nature along with: gravitational force, weak force and strong force.
[6] Maximum velocity in vacuum speed of light: According to Einstein’s Theory of Special Relativity, light in vacuum travels at a constant speed c, known as light speed. Moreover, any kind of particle cannot and will not travel in vacuum at velocities equal or greater than the speed of light c. The theory of special relativity limits signal velocity to the velocity of light in a vacuum. A faster-than-light (superluminal) signal velocity would violate causality.
[7] Superluminic speeds in water: Einstein’s interpretation an important point: nothing can move faster than velocity of speen in a perfect vacuum. Through a medium of any type (gas, liquid, or solid) light travels at a measurably slower speed. Energetic particles, are only bound to travel slower than light in a vacuum, not light in a medium. Therefore, we truly can go faster than light.
[8] Cosmic rays: They are high-energy protons and atomic nuclei that move through space at velocities close to the speed of light. They originate at sources inside or outside of our galaxy. In the impact with the Earth’s athmosphere, cosmic rays produce showers of secondary particles, such as muons.
Comments